Life on Earth
South Africa's ancient rocks bestow clues about the earliest life on Earth.
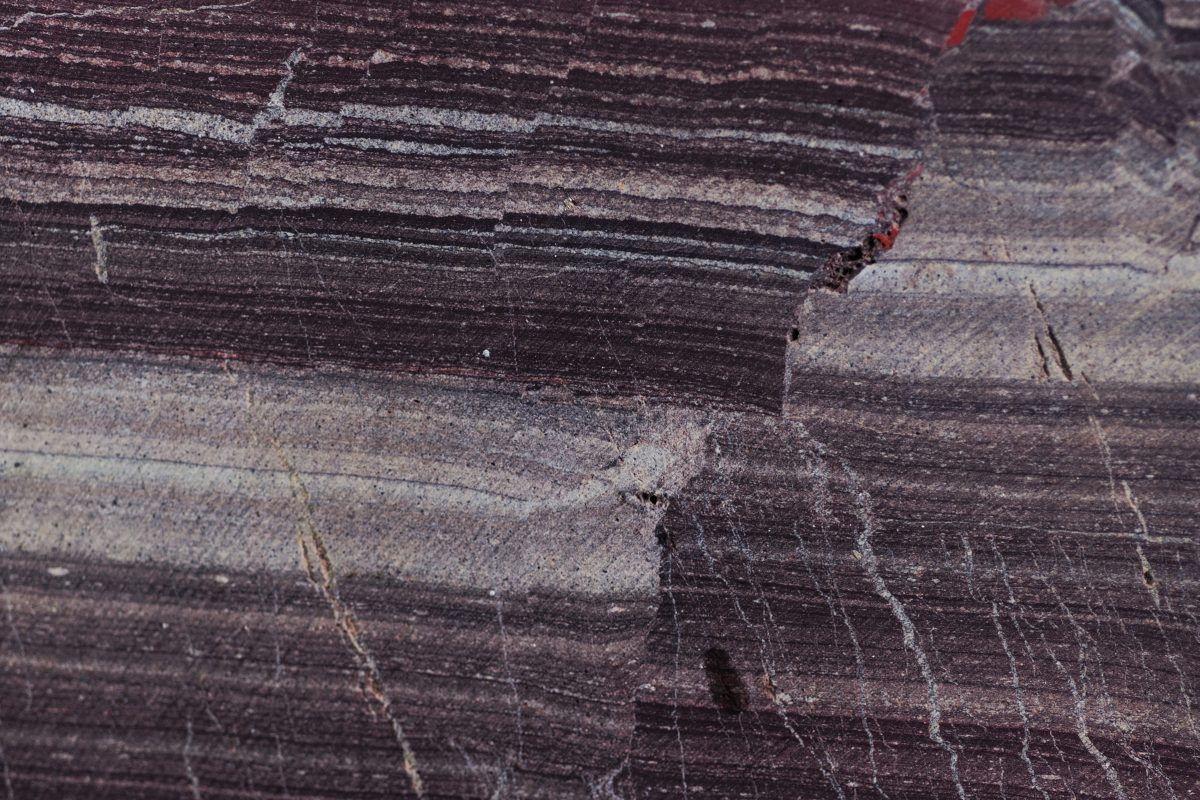
The Makhonjwa Mountains are not the tallest in the world. At 6,000 feet above sea level, the South African range more often resembles tall, green, carpeted hills. They are also not the most rugged, considered a top destination for neither mountaineers nor other high-country adventurers.
But these mountains, also known as the Barberton Greenstone Belt, own one very critical distinction: The rocks beneath them are among the oldest in the world, born on a cool and strange early Earth nearly 3.6 billion years ago.
These geologic timekeepers are one of the few places on the globe that preserve the gradual evolution of our planet, including its land composition and oceans, through a span of time difficult to fathom on a human scale. They are also one of the few places on Earth where evidence of ancient life can be found. Locked in this mountain range in South Africa are the recipes for the complexity of life we know today.
This is what brought Clark Johnson to South Africa. A professor of geoscience at the University of Wisconsin–Madison, Johnson and his collaborators around the world study Earth’s geologic past in order to better grasp when and how life on the planet began. They also hope to better understand where we are headed.
The history of life on Earth is, in large part, the history of oxygen, “because without the presence of oxygen, being able to breathe oxygen, we wouldn’t be here,” says Nicolas “Nic” Beukes, a South African geologist at the University of Johannesburg who for decades has been one of Johnson’s most important collaborators.
Oxygen, a simple gas that exists in our atmosphere as a partnership between two single oxygen molecules intimately bound together, transformed the planet from a mostly inhospitable, barren chunk of rock to a wildly diverse domicile for everything from bizarre single-celled organisms to complex animals like apes and people.
The origins of that first oxygen are part of a story Beukes and Johnson are starting to piece together, rock by rock. And it begins with an unusual collection of rock from Barberton that together they began to study in 2013.
‘You have to come down and see this!’
Much like astronomers, who can look back in time by capturing data from many light years away, geologists can peer back into Earth’s history by studying rock records that extend miles beneath the planet’s surface.
Beukes has access to ancient rocks so well preserved they reveal some of the planet’s earliest secrets. They come in the form of mining drill cores donated to him by companies in search of rich deposits of diamonds and gold, manganese and chrome. South Africa is known as much for its mining industry as its wildlife — it’s one of the most-drilled places in the world.
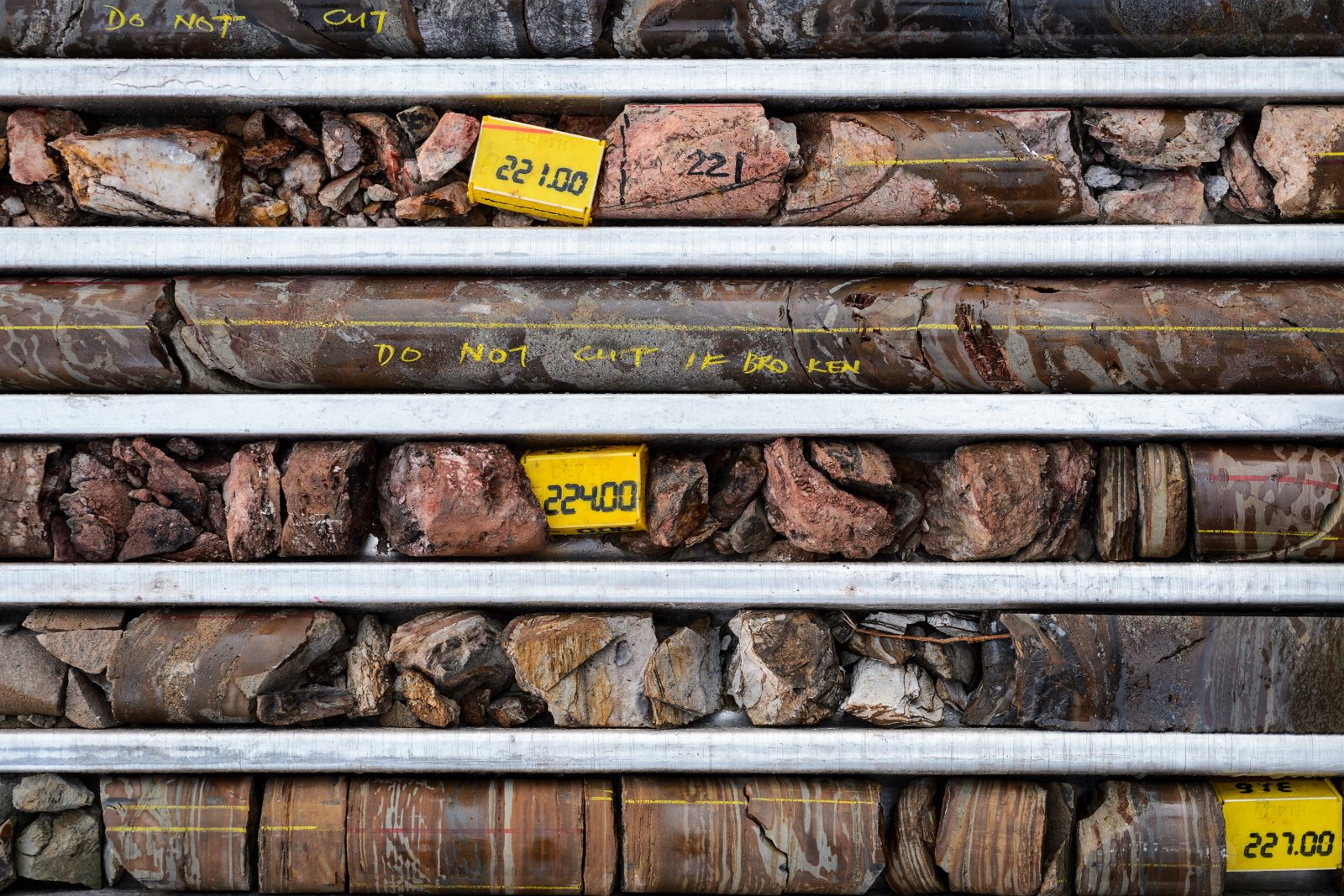
These cores offer potentially priceless scientific opportunity. While very old rocks can be found in Wisconsin and elsewhere, most have been altered over time by high temperatures, pressures and mechanical forces, known collectively as metamorphism. This may have changed or destroyed the records they might otherwise have preserved.
The cores from Barberton formed in Earth’s infancy and were largely spared from metamorphism due in part to its location on the Kaapvaal Craton, a relatively stable portion of Earth’s crust. Because they were drilled from deep underground, the rock within the cores was also spared the damage of weathering that takes place near the surface.
One such drill core from the Barberton Mountains that Beukes obtained in 2012 is marked by striking bands of color and texture. It extended to a depth of 500 meters, or nearly 1,600 feet.
“Nic had done the sedimentology on it,” Johnson says. “He told me: ‘Hey, you have to come down and see this!’”
To find life, turn over rocks
At the University of Johannesburg, tucked inconspicuously between tree-lined streets in the heart of South Africa’s largest city, Beukes and his team in the Centre of Excellence for Integrated Mineral and Energy Resource Analysis have amassed so many rock samples they now store the excess in corrugated metal shipping containers behind their building.
A self-described field geologist, Beukes is interested in scouting rocks and understanding their mineral compositions. “Nic is one of the gods of early Earth geology,” Johnson says, because much of what the world knows about early Earth geology as told through South Africa’s rock record is due to his tremendous efforts. Johnson is a specialist in the chemical story locked within these very rocks and minerals.
One of Beukes’ postdoctoral fellows, Hervé Wabo, conducted the initial analysis of one part of the Barberton core, called BARB4, with an interest in how Earth’s early magnetic field might be recorded in the rocks.
Wabo made great progress, spending most of the winter of 2013 examining the striated greens, pinks, reds, purples and grays that characterize the rock. Each pigmented band represents a sediment layer originally laid down on the bed of an ancient sea, the colors a display of the minerals trapped within layers as they formed.
At the time, no one was certain what Wabo would find.
Then he encountered a problem. Wabo noticed signatures in the magnetic character of the rock that hinted some of the core had been altered by another geologic event that occurred later.
“So we had to go to the scrappier, smaller part of the core — the deepest and hardest to pull up,” says Johnson. “That actually turned out to be the most important part.”
It was geologically and geochemically intact, dating between 3.2 billion and 3.3 billion years old, marked by alternating dark and light layers.
Johnson and his team began probing the chemical makeup of those different layers. What they found was surprising evidence about early life on Earth and the oxygen crucial to life as we know it today.
Building a planet
To understand the origins of life on Earth, it’s important to understand the origins of the Earth itself.
The story of Earth began approximately 4.6 billion years ago, when a giant cloud of molecular building blocks collapsed under the force of gravity and gave birth to the sun. The leftover material became trapped in the sun’s orbit, forming the solar system.
Earth organized around a dense core of iron as hot as the surface of the sun — more than 10,000 degrees Fahrenheit — while a liquid outer core composed of iron and other metals surrounded it. Around these layers, Earth’s mantle developed and, as the new planet began to cool, a crust solidified around its outer boundary, forming land.
When Earth acquired its oceans is less clear, though evidence generally suggests the planet became aqueous as far back as 4.3 billion years ago. Looking back now, it was only a matter of time before the oceans harbored primitive life.
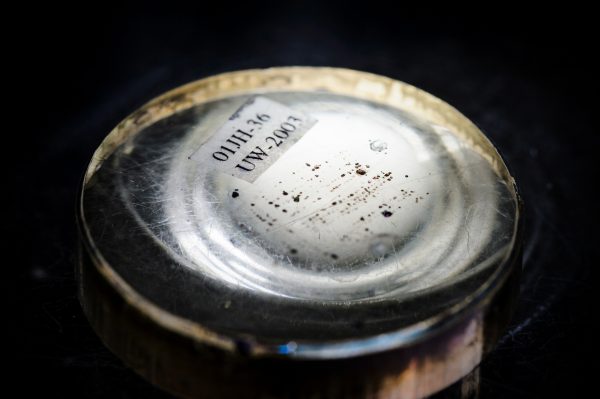
Zircon
The oldest piece of Earth’s crust ever identified is a microscopic crystal called a zircon, discovered by a team led by UW–Madison geoscience professor John Valley.
The microscopic fragment isolated from a rock outcrop in Australia is 4.4 billion years old, revealing that Earth’s crust began forming shortly after the planet was born.
In late 2017, along with a collaborator at the University of California, Los Angeles, UW–Madison geoscience professor Valley and a small team of other UW–Madison researchers confirmed the discovery of the earliest fossils of living organisms ever found on Earth, in rock from another geologically stable locale in Australia, dating back nearly 3.5 billion years.
Although older rocks have been proposed to contain evidence for life, such rocks have been heated and deformed, rendering the results more controversial.
The fossils show that Earth began to sustain life shortly after the planet formed, though it remained simple and microbial. When did life become more complex? The rocks of Barberton captured and held onto at least part of that story, inscribed with hints of oxygen.
The origins of complex life
Around 600 million years ago, just a blink of an eye by geologic time, oxygen became one of the predominant gases in Earth’s atmosphere. This coincided with an explosion of complex lifeforms in the sea, from soft-bodied jellyfish and worm-like creatures, to bug-like trilobites and five-armed starfish. Later, primitive plants began to flourish on land and animals crawled out of the oceans to commence their evolution on dry ground.
Where that oxygen came from is the story Johnson and Beukes hope to help tell. “We would actually be missing the whole story if we only focused on Earth’s atmosphere,” says Johnson, “because it was the last to be oxygenated.”
Oxygen first accumulated in the sea as cyanobacteria evolved metabolisms that allowed them to harness the energy of the sun to break down water molecules and utilize the energy to survive. Oxygen was a byproduct.
“This is the origin of where we came from,” says Beukes. “These are the origins of the organisms that started to breathe oxygen, the deep origins of humankind.”
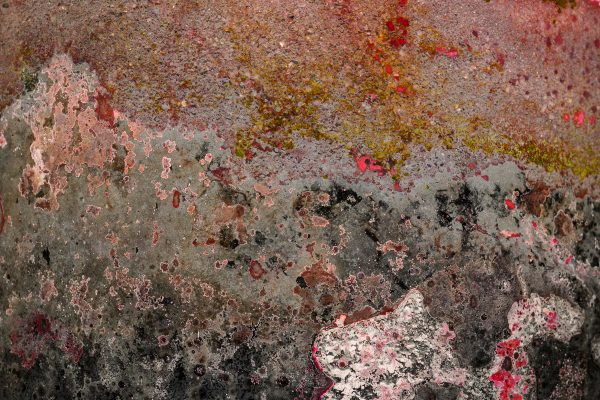
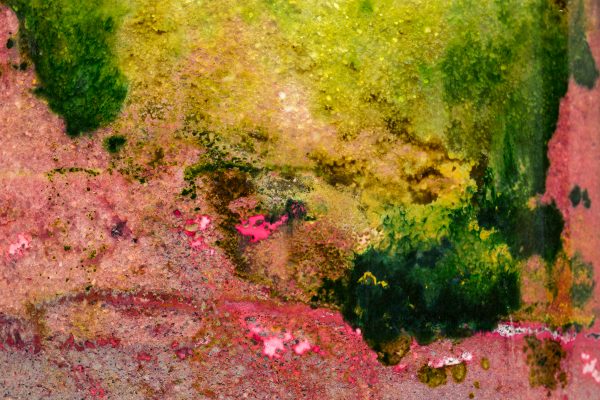
That metabolic conversion, Johnson says, was a challenging one to accomplish because the energy required to break apart the bonds of a water molecule is great. But the payoff — a food source for the microbes — is even greater. It paved the way for complex life.
The layers tell the story
You don’t have to be a geologist to notice the difference in the layers that make up the Barberton core.
“Some layers have darker color, some pinkish, some lighter gray, made up of little granules of iron washed in from somewhere in the shallow part of the ocean,” Beukes explains. “Clark and his students discovered that these lighter (pink) layers have a different composition from ones that formed in deep water.”
The researchers determined that the lighter layers must have originated in a flat and shallow basin of water on a shelf of the continent that dropped down into a deeper sea. Through wave action or some other disturbance, the sediments in this basin settled onto the deep ocean floor, eventually compressing into the rock extracted by miners more than 3 billion years later.
The deep layers also contained iron, which originally came from deep within the Earth via seeping hydrothermal vents, or seams, on the ocean floor.
But the light pink layers, Johnson and his research team found, contained iron that had partially oxidized before it was laid to rest on the bed of the ancient sea.
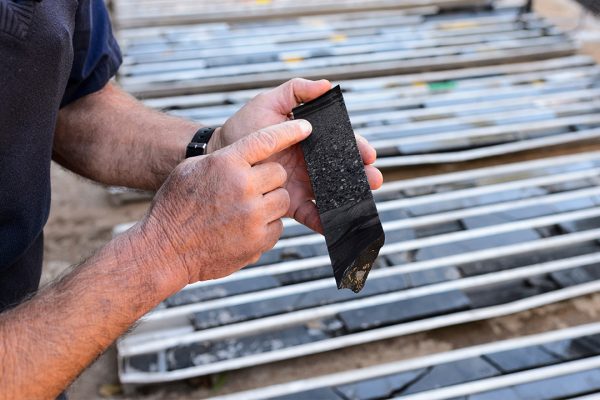
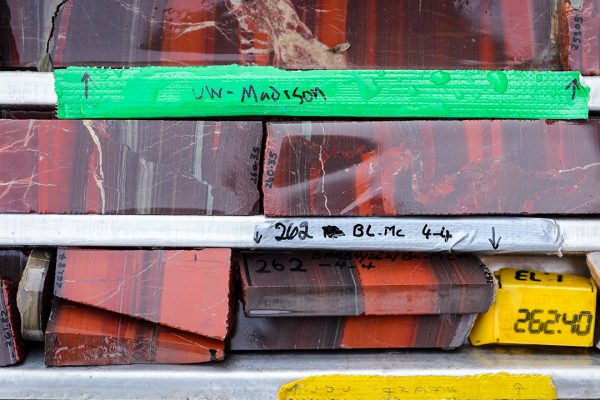
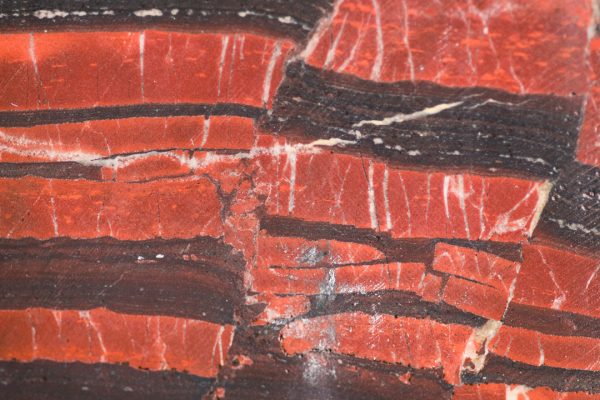
Most of us know iron oxidation as rust, the chemical process that plagues vehicle owners after a long, salty winter and turns shiny nails in a bucket of water a dull orange-red. It happens when iron gives up its negatively charged electrons to another element which greedily grabs them up, changing the character of the iron itself.
This finding intrigued Beukes, Johnson and the rest of the team, because iron oxidation can occur when the element encounters oxygen. It hinted that oxygen may have been present in the shallow seas where the sediments in the lighter layers originated.
So Johnson and his team searched for other clues to help them solve how the iron was oxidized. They looked within the layers for the presence of another element, uranium, which would only have been trapped in the rock if oxygen was there, too. And they looked at the element thorium, which would tell them how fast or slow the sediment accumulated on the sea floor.
Locked within the light pink layers, which sedimented quickly and possessed partially oxidized iron, was the giveaway: uranium. The element was not present in the darker layers, which accumulated more slowly.
The rock revealed a story: At least as far back as 3.2 billion years ago — and likely longer — there was oxygen in the shallow, early seas. It could only have been produced by living organisms — in this case, microbes known as photosynthetic cyanobacteria.
“This actually proves oxygen was available in shallow water,” says Beukes. “We didn’t know it was this exciting until Clark did his sophisticated analysis and we said: ‘See, this is what a rock can tell you.’”
The most important biological innovation
For life on Earth, this oxygen was just the beginning.
It took much longer — another billion years — for oxygen levels to increase in Earth’s atmosphere. That, too, is because of iron.
Early on, oxygen exhaled by microbes hit the copious iron in the seas and on land. The gas and the iron rapidly interacted through oxidation. It wasn’t until this free iron was exhausted, absorbed like water in a sponge, that oxygen could begin to accumulate in the atmosphere.
“It took a long time for oxygen to rust the whole world,” says Beukes.
That began a bit less than 2.5 billion years ago, when atmospheric oxygen levels spiked in a phenomenon scientists call the Great Oxidation Event. Eventually, oxygen became a major component of Earth’s atmosphere.
“Once that happened, life radiated quickly because it outcompeted everything,” says Johnson. “It was the most important biological innovation on the planet.”
That set in motion an evolutionary chain of events that ultimately led to the origins of modern humans, roughly 200,000 years ago.
“It’s important to understand the history of oxygen on Earth,” says Beukes. “It’s where we come from.”